What is the energy intensity of fiber optic cables? Our best estimate is that moving each GB of internet traffic through the fixed network requires 40Wh/GB of energy, across 20 hops, spanning 800km and requires an average of 0.05 Wh/GB/km. Generally, long-distance transmission is 1-2 orders of magnitude more energy efficient than short-distance.
An optical fiber consists of a glass core, through which light signals can travel ultra-rapidly via total internal reflection, surrounded by at least one sheathing layer.
A fiber optic cable consists of at least one optical fiber, often many, surrounded by protective exterior layers of sheathing and possibly armoring. By 2020, over 5bn kilometers of fiber-optic cables have been deployed globally. Estimates vary, but the fiber optic cable market is likely worth $10bn per year.
A transceiver (aka a transducer) is an electric device that converts electrical signals into light signals (e.g., a laser-based transmitter) or vice versa (e.g., a photo-diode based receiver). The optical transceiver market is worth around $10bn per year.
The fiber optic network is a constellation of transceivers and fiber optic cables, which are capable of transmitting data between data-centres and internet users. A commonly used acronym is PON which stands for the Passive Optical Network, and simply transmits data.
Bitrate is the capacity of a digital network to transmit information. It is measured in Gbps. Gbps stands for Gigabits per second. 1 Gbps means that 1 billion bits of information can be passed along the fiber optic cable each second (there are 8 bits in a byte).
The frequency of a fiber optic system is measured in MHz. 1 MHz means that the cable can carry 1 million distinct ‘packets’ of information per second (i.e., 1 Mbps). Typical frequencies are 10-100MHz, but can reach into the GHz range.
Many distinct signals can be carried through a fiber optic cable at the same time by “multiplexing” them. This might include carrying them at different frequencies or wavelengths. For example, ‘dense wavelength division multiplexing’ (DWDM) can carry 80-100 different frequencies through the same optical fiber (different colors). The signals can later be de-multiplexed.
Prysmian notes a typical fiber optic cable will enable 1-10 Gbps download speeds, which is 30-50x faster than a comparable copper cable (25-300 Mbps), and an order of magnitude above satellites or DSL (0.5 – 75 Mbps). The world record for data transmission through a fiber optic cable at the time of writing is 1.84 petabits per second (achieved in 2022 by researchers from the University of Denmark, on a single, 7.9km fiber optic cable, split into 37 lines with 223 frequencies per line). This is something equivalent to transmitting 1bn Zoom calls simultaneously.
The strength of a signal in a fiber optic cable is measured in dBm, where 0 dBm is the equivalent of 1mW (1,000th of a Watt). Note that decibels are logarithmic around base 10. Hence 10dBm is equivalent to 10mW, 20dBm is equivalent to 100mW, 30dbm is equivalent to 1W; while -10dBm is 0.1mW, -20dBm is -0.01mW and -30dBm is -0.001mW.
Attenuation is the difference between the launch power of the signal from the transmitter and the power of the signal at the receiver. The rate of attenuation will depend on the precise dimensions and materials of the cable, but a good rule of thumb is in the range of 0.2dB per kilometer. Each connector likely also introduces a 0.75dB loss.
To combat the impacts of attenuation across a longer cable one must either: project a higher power level from the initial transmitter; deploy a more sensitive (and thus more expensive) receiver; or install a series of amplifiers/repeaters along the length of the cable, to boost it (e.g., every 20km). Although this adds to the energy intensity of fiber optic cables.
Another limitation on the length of a cable comes from dispersion. This is not related to the signal attenuating (i.e., getting weaker). It is due to the signal ‘spreading out’ and becoming noisy. To combat attenuation, filtering needs to be applied within the circuitry of the amplifier/repeater. As a general rule, thin “single mode” fibers, with c10ฮผm cores will have longer ranges than thicker “multi-mode” fibers with c50-100ฮผm cores, as the thinner core confines the light more and limits dispersion. So in a sense, a fiber optic cable is the opposite of a pipeline, where greater widths enable greater flow.
Using these definitions, we can compile data into the energy consumption of fixed fiber lines and their bit rates. Using these numbers, we can estimate the power consumption of data transmission infrastructure, which is ‘always on’, transmitting signals on one side of a fiber optic cable and listening for signals on the other side.
Power consumption of fiber optic cables can range from 0.01-100 W/Gbps depending on the length of the cable (chart below). As a mid-point, a 2-5km cable might have a power consumption of 1W/Gbps and consume around 0.1 Wh/GB of data transmission, which equates to 0.05 Wh/GB/km. Numbers can be flexed in the data-file.
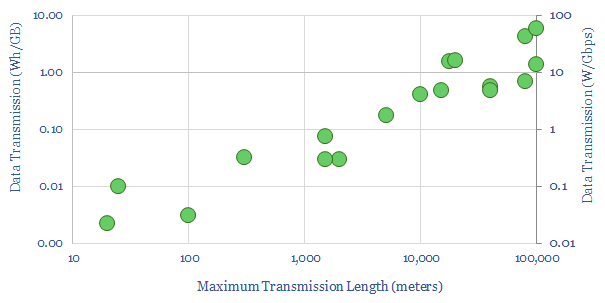
Larger and more highly utilized cables will have 1-2 orders-of-magnitude lower energy consumption (chart below). Thus the energy intensity of fiber optic cables is not a fixed number, but highly context-sensitive.
Energy consumption will continue falling, per the historical trend (chart below, data here). The energy in the signal that is transmitted through a fiber optic cable (quoted in dBm), in physics terms, represents 0.05% of the total electricity use of the data transmission network. The energy consumption is not in the laser pulse. It is in encoding and decoding it, and the balance of electronic systems. Hence there is huge room to improve, including through improved cables, improved transceivers, more sensitive photo-diodes, greater frequencies, greater multiplexing.
Overall the energy consumption of a fiber optic cable is very low. It might take 0.05 Wh to move 1GB by 1km. For contrast, the numbers to move 1 ton or 1 person by 1km can be around 15,000x higher (data here).
Our outlook on the future energy consumption of the internet is written up in our recent research note here, and all of our broader energy demand data are here.
Corning is the leading manufacturer of fiber optic cables and had produced over 1bn kilometers of optical fiber by 2017 (and comes up in our glass fiber research). Prysmian produces 30M km of optical fiber each year across five plants worldwide (and comes up repeatedly in our research). Many sources cite Finisar, a division of Coherent and Molex as having the largest market share in transceivers. Broadcom is a $260bn (market cap) giant, producing connectivity equipment, and has a Top 3 market share in transceivers. Sumitomo is also active, making both cables and transceiver modules. Air Products notes that it supplies industrial gases, such as argon, helium and hydrogen used in production. High-quality silica glass and specialty plastics are also used in the cabling.