Industrial energy efficiency is basically impossible to define or measure. This means economy-wide CO2 prices may be the most effective way to incentivize efficiency gains, whereas specific policies may miss the mark. This note illustrates the argument, in the context of ‘home cooking’ a process technology with which most readers are likely familiar…
Improving energy efficiency is crucial to decarbonizing the global energy system and explains 20% of the bridge towards ‘net zero’ in our research (chart below).
Our recent research has also focused on the topic of energy efficiency in the context of industrial heating, arguing that granular and case-specific efficiency gains are needed, rather than over-simplified statements such as “electrify everything” (below).
But what is efficiency? Measuring the efficiency of an energy-consuming process is conceptually complex. In order to illustrate the complexity, this note will consider efficiency in home-cooking. It is a process with which we are all familiar (more so than, say, ethane cracking, below).
Ideally you will also come away from this article with some interesting insights for the next time you are in the kitchen. Cooking is responsible for 4.5% of a homeโs energy use, according to the DOE, and this number excludes refrigeration or dishwashing electricity. So efficicency gains here cannot hurt either.
Standard tests for measuring cooking efficiency?
The first way to measure the energy efficiency of heating technologies is to estimate the percent of incoming energy that is converted into heat. The problem with this definition is that all combustion technologies and resistive heating technologies score close to 100% efficient. Combusting gas on a gas stove released almost all of the energy in the gas as heat (and a very very small portion of light). Passing a current through the electrically resistant nichrome coil also releases almost all of the energy as heat. But as we will see below, it would be grossly incorrect to use this definition and label cooking as 100% efficient.
Instead, one of the DOEโs standard tests for the efficiency of a cookstove technology is to place a solid aluminium test block on a stove on maximum power, until the temperature in the block has risen by 144ยฐ F (80ยฐ C). Then the the heat is reduced to 25% of maximum and held for 15-minutes. Efficiency is calculated as the ratio of thermal energy absorbed by the block divided by the energy consumed by the cooker.
On these heat-up tests, typical results might be that an electric stove would achieve 70-80% efficiency and a gas stove might achieve 30-40% efficiency. Burning gas is less efficient at heating up the aluminium block, as a large portion of energy from the flame heats the surrounding air, which then convects defiantly around the kitchen. However, as long as the pan covers the entire electric coil, electric stoves do not suffer this drawback.
But a similar problem may already have occurred in the power station that generated the electricity powering the electric stove. If that power station was only c40% efficient at converting natural gas energy into usable electricity, a reasonable number (data below), then the total efficiency of the electric stove will be 25-30% overall, and actually less efficient than the gas stove, once the electricity generation is considered.
Is the waste heat really wasted? If it is a cold day, you might regard the energy leaked into your kitchen by the gas (or electric) stove as “useful” after all, reducing the load on your home heating system. However, if it is a hot day, it may be exacerbating the load on your air conditioner, hurting efficiency further. Similarly, if the cooker is close to the fridge, waste heat may be increasing refrigeration loads.
Another problem with the aluminium heat-up test is that it cannot be used to test induction heaters, which induce eddy currents in ferromagnetic cooking vessels. Those currents generate heat in proportion to the electrical resistivity of the cookware (by the Joule effect). But aluminium blocks are non-ferromagnetic. They are not heated up by electromagnetic induction. Hence the standard test need to be modified, placing the aluminium blocks in ferromagnetic pans. Because induction heaters generate their heat directly in the pan, their efficiency is usually 80-90% on these modified tests.
The biggest problem with the heat-up test is that most people do not eat aluminium blocks for dinner. Hence, one technical paper measured the energy requirements to boil 200g of potatoes, in 200ml of water and a 700g stainless steel pan, obtaining the CO2 and cost profiles below. This further complicates our assessment of efficiency. The ultimate goal here is to produce cooked potatoes, not to produce hot pans and hot water (which will ultimately be poured down the sink). Since the potatoes comprise just 20% of the mass of the system being heated, the efficiency of this potato cooking process cannot be more than 20%. We estimate 9% efficiency in the gas cooker, 14-16% in the electric cooker, and c10% in the induction cooker (although this was not a fair test, as the induction cooker was run at a higher overall heat rate, saving time, but using more power).
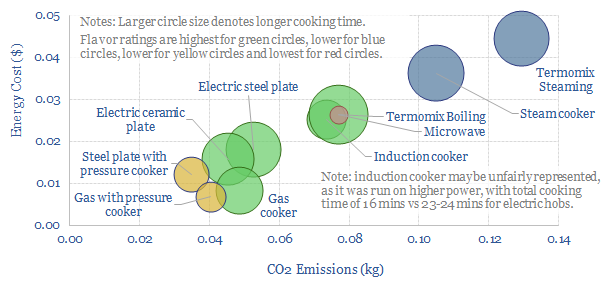
This also highlights that efficiency is a function of behaviour. Cooking faster wastes more heat. Cooking half (or double) the number of potatoes in the pan would have approximately halved (or doubled) the process efficiency. As would using a 2x larger (or 50% smaller) pan. Simply covering the pot with a lid lowers the energy consumption by 12-16%. Specialized cooking equipment also helps, as boiling water in an electric kettle uses 50% less energy than in a pot on an electric stove. A pressure-cooker slashes stovetop energy by 50-75%. An egg cooker may use 60% less energy than boiling eggs in a pot. And a rice cooker can use 77% less energy versus cooking rice in a pot. Ultimately, this means that cooking behaviours are much more important to overall cooking energy and CO2 emissions that whether you are cooking on a gas or electric stove top.
Another efficiency question-mark is the risk of over-cooking or burning food. Technically, in these cases, the heater has efficiently transferred heat into the food, but it was not useful heat in the sense of achieving a desired outcome. If the dish is ruined, then all prior heat transfer now needs to be “written off” in your efficiency calculation. This is where electric cooktops are more prone to problems. The heating element holds large quantities of heat and is relatively unresponsive to changing the heat rate. In one study, induction cookers can be set to very precise temperatures, gas burners tended to overshoot desired temepratures by 1C, while electric cookers tended to overshoot the desired temperature by 2-5C, and then take several minutes to cool back down again. Induction cookers also have the advantage of being less likely to cause burns to people (as long as you are not made of ferromagnetic metal).
The final question mark is whether qualitative factors matter for efficiency. Back to the potato test, noted above, cooking times ranged from 6 minutes in the microwave through to 26-minutes in a Termomix. The best flavor and texture was assessed to come from the boiled potatoes, while the worst was in the microwave. However, soluble nutrients such as vitamin C and potassium are actually lost when cooking in water, meaning the final product is debatably less nutritious. Surely no analyst can factor these subtleties into their efficiency calculations.
Why this matters: industrial efficiency is complex?
Our recent research has noted a very wide range in useful energy efficiencies of industrial heating technologies (data below). The landscape is so complex that we concluded the only way to maximize industrial efficiency was to avoid over-simplified maxims such as “electrify everything” and impose economy-wide CO2 prices, which will incentivize individual process engineers to explore ways to boost efficiencies and lower CO2 emissions.
If you are willing to grant the complexity around measuring efficiency in a process as simple as cooking potatoes, I can promise you, it is more complex in the industrial production of steel, cement, plastic, glass or paper products. All of which are explored in our note below.
Analogies can nevertheless be drawn from our cooking examples above. For example, there is a risk that efficiency mandates are as strangely detached from actual operating conditions as the aluminium block test above, or similarly that they cannot be implemented in particular sub-industries. Specific efficiency tests could be prone to being gamed at the expense of real-world efficiency. Another risk is that forcing the phase-out of one fuel in favor of another may not drive any decarbonization, if the underlying problem is inefficient processes and behaviours.
Now imagine you are trying to regulate the energy efficiency of cooking. This is a genuine question and challenge to anyone reading: what specific policies would you implement as a regulator to improve the efficiency of people cooking in their kitchens? Personally, I can think of a lot of bad answers, and only a few good ones (maybe a tax credit for taking an interactive educational course into energy efficient cooking behaviours?).
Industrial efficiency is just as complex and just as challenging to improve with specific policy measures. Again, this is the argument for a flat, fair and generalized CO2 price, incentivizing each industrial facility to seek efficiency gains where they can.
Which cooking technologies are lowest cost and lowest carbon?
Our own attempt to estimate the costs and CO2 intensities of different home cooking systems is show below. At a CO2 intensity of 0.35kg/kWh across the grid (reflecting c20% renewables penetration), we estimate a gas cooker is c15% higher carbon overall, in a household where heating and air conditioning loads balance out across the year. Gas and electric stoves have comparable costs. Induction stoves may be more powerful, sensitive, and professional, but they are likely 2x more expensive, when reflecting the $1.2-2.4k purchase prices on leading units from Frigidaire, GE and Samsung. Their payback periods on energy bills are estimated at around 44-years in one paper.
Numbers and data-points from underlying technical papers can also be stress-tested in the data-file above.
Sources
Das, T., Subramanian, R., Chakkaravarthi, A., Singh, V., Ali, S. & Bordoloi, P (2006) Journal of Food Engineering 75 pp 56โ166
Korzeniowska-Ginter, R. (2019). Energy consumption by cooking appliances used in Polish households. Conf. Ser. Earth Environ. Sci. 214 012096.
Livchak, D., Hedrick, R. & Young, R. (2019). Residential Cooktop Performance and Energy Comparison Study. Frontier Energy Report # 501318071-R0
Sweeney, M., Dols, J., Fortenbery, B. & Sharp, F. (2014) Induction Cooking Technology Design and Assessment. Electric Power Research Institute, ACEEE Summer Study on Energy Efficiency in Buildings.